​Severe Acute Respiratory Syndrome Coronavirus 2
COVID-19 caused by SARS-CoV-2 emerged in December 2019, Wuhan, China. Common symptoms are fever, cough, shortness of breath, fatigue, loss of smell or taste, muscle or body ache, running nose, nausea, etc [1]. 60.8 million people are infected worldwide and more than 260 thousand people died in the U.S [2].
Photo Credit: Veronica Falconieri Hays; Source: Lorenzo Casalino, Zied Gaieb, and Rommie Amaro, U.C. San Diego (spike model with glycosylations)
Introduction
Structure of SARS-CoV-2
​
SARS-CoV-2, a member of the beta group of coronaviruses family, is an enveloped positive-sense single-stranded RNA virus. Beta coronaviruses infect only mammals and cause respiratory illness in humans and gastroenteritis in animals [3]. SARS-CoV-2 has spike protein (S), envelope protein (E), membrane protein (M), nucleocapsid protein (N), and Hemagglutinin-Esterase dimer (HE) on its surface [4].

Fig. 1. Structure of SARS-CoV-2 that causes COVID-19, including E protein, M protein, S protein, and N protein.
​​Figure 1 shows a graphic depiction of the structure of SARS-CoV-2.
-
S protein: S protein is cleaved by TMPRSS2 (cellular furin-like protease) into two polypeptides, S1 and S2. S1 attaches to ACE2 (angiotensin-converting enzyme 2), and S2 facilitates the fusion of the viral and cellular membranes. [4]
-
E protein: A transmembrane protein functions as an ion channel, allowing pathogenesis by the assembly and release of virions. [4]
-
M protein: The transmembrane domain may contain a T cell epitope, which causes cellular immunogenicity. M protein can also neutralize antibodies produced in patients. [4]
-
N protein: A phosphorylated protein allows the viral RNA to form a ‘beads on a string’ conformation. [4]
-
Hemagglutinin-Esterase dimer (HE): It binds to sialic acids on the host cell surface membrane, and assists the release of virus from infected cells. [4]
​
Origins of SARS-CoV-2
​
SARS-CoV-2, a member of the beta group of the coronaviruses family, highly resembles SARS-CoV that caused severe acute respiratory syndrome (SARS) in Guangzhou, China in 2003. Simplot analysis revealed that SARS-CoV-2 was highly similar to the genome of BatCoV RaTG13, with an overall genome sequence identity of 96.2%. SARS-CoV-2 and SARS-CoV belong to the same species, SARSr-CoV-2, as the amino acid sequences of seven conserved domains in ORF1ab that are used to classify coronaviruses species were 94.4% identical between SARS-CoV-2 and SARS-CoV [5]. Analysis of the receptor-binding motif (RBM), that interacts with ACE2, revealed that most amino acid residues essential for ACE2 binding by SARS-S were conserved in SARS-2-S [6]. Therefore, it can be concluded that SARS-CoV-2 originated from bat coronavirus and use the same receptor for host cell entry as SARS-CoV.
​
Lifecycle
Initially, it was suspected that snakes were the possible host, however, genomic sequence analysis of SARS-CoV-2 with different bat coronaviruses supported the statement that bats were the key intermediate hosts indicated in Figure 2 [3]. SARS-CoV targets pneumocytes and lung macrophages in the lower respiratory tract, where ACE2 is abundant, consistent with its limited viral transmission. In contrast, SARS-CoV-2 replicates primarily in upper respiratory epithelia, which explains the high efficiency in shedding and viral spread [9, 10].
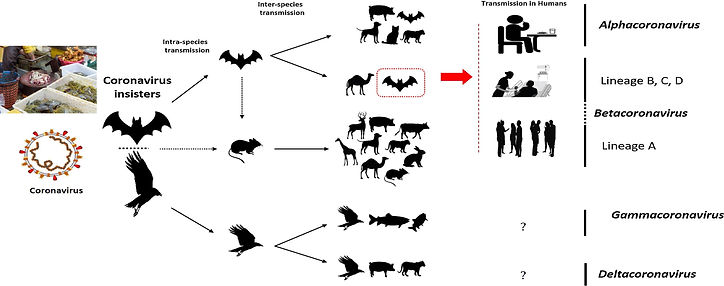
Fig. 2. The potential intermediate hosts and mode of transmission of coronaviruses (hosts of SARS-CoV-2 are cycled in red). Only α and β coronaviruses infect humans. Eating infected animal is the major cause of animal to human transmission. The virus is then transmitted to healthy individuals via close contact with an infected person. The dotted black arrow shows a potential viral transfer while the solid black arrow represents a confirmed transfer.
SARS-CoV-2 containing aerosols enter the nasopharynx by attaching to angiotensin-converting enzyme 2 (ACE2) that are abundant on surfaces of epithelial cells of the nasal and oral mucosa, which suppresses the sense of smell and taste. Then the virus passes down to the lungs, the primary site of infection. The host cells endocytose the virus that then uses the host cellular machinery for replication, transcription, translation, gene modification, assembly, and release of the virus to other cells that have ACE2 receptors, including the brain, lungs, heart, vascular endothelium, kidney, small intestine, colon, and testis [4, 7]. Therefore, the infection begins with acute respiratory distress syndrome, followed by a cytokine storm that is a potential cause of multiple organ damages and even mortality [3, 4]. Figure 3 outlines an overview of the lifecycle of SARS-CoV-2.

Fig. 3. SARS-CoV-2 life cycle showing binding, membrane fusion, translation/replication, viral assembly, and virion release.
Cell Entry
Spike protein is a homotrimeric class I fusion glycoprotein that is composed of two functionally distinct parts, S1 and S2. Receptor-binding domain (RBD) in the surface-exposed S1 bind to ACE2 on the host cell surface. The transmembrane S2 domain contains heptad repeat regions and the fusion peptide, which facilitates the fusion between viral and cellular membranes upon extensive conformational rearrangements [8]. The proteolytic cleavage of coronavirus S proteins into S1 and S2 by a proprotein convertase furin and cell surface serine protease TMPRSS2 is essential for viral uptake and fusion at the cell membrane [6,8].
​
RNA translation and replication
SARS-CoV-2 encodes 27 proteins that are crucial in virus replication and assembly [11], which include 4 structural proteins, 16 non-structural proteins (NSP1–NSP16) that encode the RNA-directed RNA polymerase (RdRp), helicase, and other proteins required for viral replication, and 7 accessory proteins (ORF3a–ORF8) whose functions are uncharacterized [12].
After being endocytosed by the host cell, viral RNA is released into the cytoplasm and two opening reading frames, ORF1a and ORF1b are translated, producing two polyproteins pp1a and pp1ab, which are processed into 16 NSP complexes that form RNA replication and transcription complexes. The function of each NSP is illustrated in Figure 4. Among the NSPs, NSP12 (RNA dependent RNA polymerase), NSP13 (zinc-binding helicase), NSP14-16 complex (mRNA capping), NSP14 (RNA proofreading), NSP15 (uridylate-specific endoribonuclease activity), and NSP7–NSP10 are essential for RNA synthesis and viral transcription [4].
​
​
​
​
​
​
​
​
​
​
​
​
​
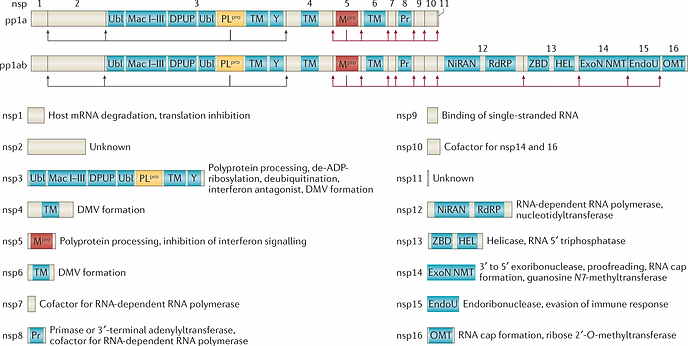
Fig. 4. Functions of NSPs.
Polypeptides translated from the genomic RNA are processed by proteases, such as 3CLpro and PLpro, into nonstructural proteins for the viral replication and packaging of a new generation of viruses. Full-length positive-sense genomic RNA is used by RdRp as a template to synthesize a full-length negative-strand RNA template that is used for genome replication and subgenomic negative-sense RNAs (–sgRNA) to produce the subgenomic mRNAs (sg mRNA) [4, 8]. Translated structural proteins mature at endoplasmic reticulum (ER) membranes or Golgi apparatus. Finally, virions are released from the infected cell by exocytosis [8].
​
Viral Release
There are three ways for a virus to be released from an infected cell -- budding, exocytosis, or cell death. For undeveloped virions, budding occurs. Nucleocapsid protein engages with the host cell membrane into which glycosylated viral envelope proteins are inserted. The host cell membrane wraps around the virion and then the membrane connecting the virion is constricted and severed to release the enveloped particle [4, 14]. Viruses that are matured at the ER or Golgi apparatus are released via exocytosis. The virus sometimes destroys the host cell machinery leading to the release of lysosomes, which results in cell death and a consequent release of virus particles [15]. Virions can either infect other cells or shed into the environment via respiratory droplets. [4].