Immune Evasion and Virulence
Many features of coronaviruses that help them to evade immune responses are still unknown, including SARS‐CoV and MERS‐CoV. Some information about immune evasion of SARS-CoV-2 is inferred from SARS‐CoV and MERS‐CoV because genes that contribute to their virulence are highly similar. The virulence factors of SARS‐CoV‐2 are mainly used to escape immune detection or to manipulate host immune responses, including delaying of interferon (IFN)‐mediated protection and the production of neutralizing antibodies or exploiting host cell machinery favoring viral replication [20].
1. Unique Furin cleavage site (FCS) in SARS‐CoV‐2
A recent study by Johnson et al. showed that a SARS‐CoV‐2 mutant without FCS in S protein had a reduced replication in Calu3 cells, and its pathogenesis had attenuated in a hamster model, which suggests that FCS may have contributed to SARS CoV 2 virulence [22]. However, the mechanism of how FCS provides advantages to SARS‐CoV‐2 needs to be explored in detail. [20]
2. Escape of immune detection
Coronaviruses evade immune responses by restricting PRRs activation. SARS-CoV and MERS-CoV prevent their RNA genome from detection by cytosolic (RIG-I) and endosomal (TLR3/7) PRRs by replicating in double-membrane vesicles that excludes these PRRs. Studies have shown that NSP1 from SARS‐CoV‐2 bound to the 40S ribosomal subunit, shutting down the mRNA translation both in vitro and in cells. Moreover, structural analysis of reconstituted NSP1‐40S and native NSP1‐40S and NSP1‐80S complexes reveals that the NSP1 C terminus obstructs the mRNA entry tunnel, thereby effectively blocking retinoic acid‐inducible gene I (RIG‐I) and interferon‐stimulated genes (ISGs) [20]. These two genes regulate innate immune responses against SARS-CoV-2 infection, so blocking them can effectively evade immune responses. In addition to physically excluding PRRs from the site of viral replication, CoVs also suppress PRR signaling. For example, SARS-CoV suppresses TLR-associated signaling molecules in infected macrophages, although the specific mechanism and its net effect on TLR signaling are undefined. [20]
3. Manipulation of host immune response
SARS-CoV-2 triggers a delayed but hyperactive innate immune response, a cytokine storm, which leads to an attenuation of T cell-mediated antiviral functions and tissue damage. Several innate immune signaling proteins are targeted by SARS-CoV-2 viral proteins. For example, the IFN pathway is targeted by Nsp13, Nsp15, and Orf9b. The NF-κB pathway is targeted by Nsp13 and Orf9c [12]. These proteins influence the normal functions of the Type I IFN pathway, either by inhibiting transcription or by acting on effector mechanisms [15]. Patients with severe COVID‐19 show a reduced IFN (type I and III) response but high levels of cytokines. The suppression of early IFN response is controlled by ORF3b and SARS‐CoV‐2‐PLpro. SARS‐CoV‐2‐PLpro cleaves the ubiquitin‐like protein ISG15 from IRF3, significantly reducing type I IFN response. In addition, SARS‐CoV‐2 infection of lymphoid tissue causes loss of germinal centers and depletion of Tfh cells, and a specific population of B cells—Bcl6+—which are involved in antibody formation [20].
After entering the cell, coronaviruses encode different proteins that interact with signaling molecules of TLRs and the JAK-STAT pathway. MERS-CoV encoded matrix protein, accessory proteins from ORF 4a, ORF4b, and ORF5, which inhibit the IFN promoter and nuclear localization of IRF3. PLpro, encoded by SARS-CoV and MERS-CoV, prevents the dissociation of NF-κB, while nonstructural proteins of SARS-CoV (PLpro and ORF3b) inhibit IRF3 phosphorylation and therefore its translocation to the nucleus. These viral proteins also inhibit the JAK-STAT pathway, resulting in the inhibition of genes by ISRE promoters (Figure 9). An overall decrease in the transcription of antiviral genes is observed in patients with COVID-19 infection because of lower levels of Type I and III interferons with high ISG expression and chemokine secretion. Therefore, a decrease in the innate antiviral response, along with hyper-inflammation, could be one of the causes of COVID-19 severity [17].

Fig. 8. SARS-CoV-mediated evasion of host innate immune response. RIG-I, Retinoic acid-inducible Gene I protein; MDA5, Melanoma Differentiation-Associated protein 5; MAVS, Mitochondrial antiviral-signaling protein; M, Membrane protein; N, Nucleocapsid; IFNAR, IFNα/β receptor; ISGs, IFN-stimulated genes; ISRE, IFN-stimulated response element.
4. Hijack host cell machinery
SARS‐CoV‐2 mimics an epithelial ion channel to hijack host cell machinery. The amino acid sequence of the S1/S2 cleavage site of S protein of SARS‐CoV‐2 has a high similarity to the Furin‐cleavable peptide on the human epithelial sodium channel α‐subunit (ENaC‐α). The structural decoy of the S protein to ENaC‐α allows the virus in the lungs to compete for furin and thus inhibiting proteolytic activation of ENaC‐α, which reduces the fluid clearance from alveolar cells (Figure 9). ENaC‐α malfunction may be aggravated with virus‐induced cell injury, immune cell infiltration, and release of inflammatory cytokines/chemokines that consequently results in ARDS [20].
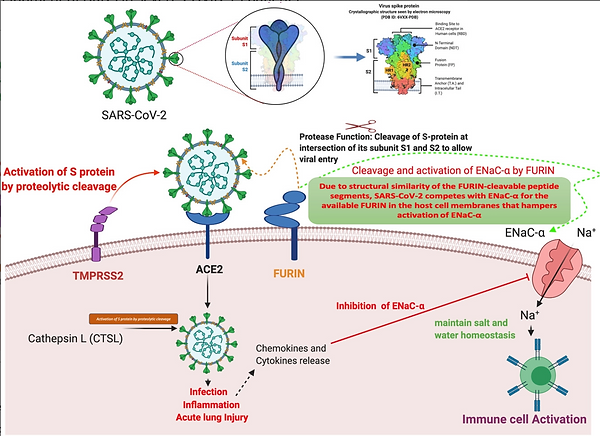
Fig. 9. SARS‐CoV‐2 mimics an epithelial ion channel to hijack host cell machinery.